AGUSTI LAB
​
Research
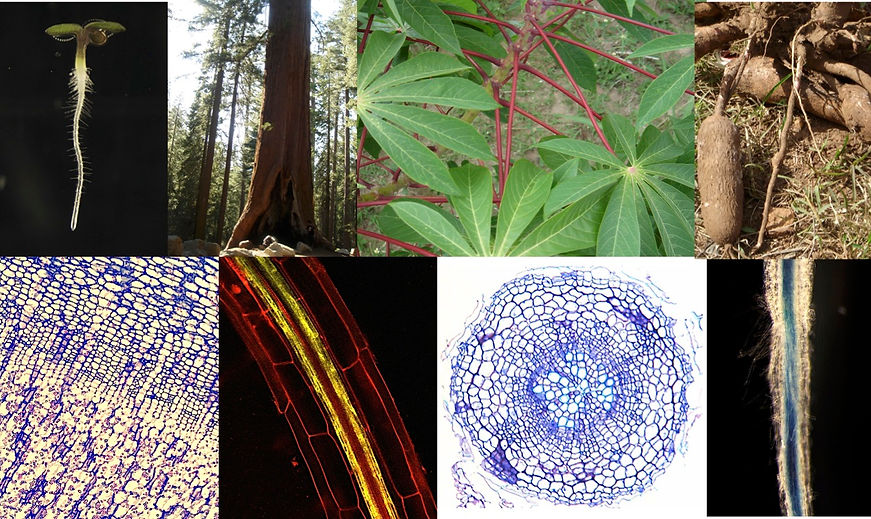
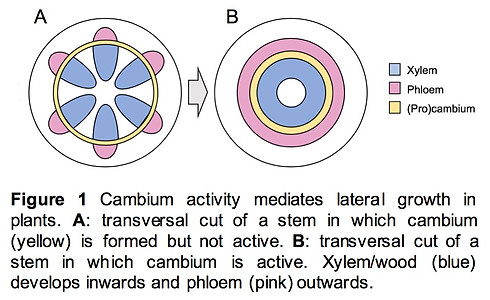
Conceptual Framework
Multicellular organisms develop by coordinating and integrating multiple growth programs. In plants, radial growth is the process by which stems and roots grow in girth. This is a pivotal process in development that provides plants with the required mechanical support and stability that they need to expand their growth and sustain their structures. Radial growth is mediated by the activity of a pool of stem cells collectively termed cambium. The cambium is found in the shape of a ring that localises internally to roots and stems, and is specialised in developing, exclusively, the so-called secondary vascular tissues: secondary xylem towards the internal part of the plant and secondary phloem towards the external part of it (Figure 1). The accumulation of concentric layers of xylem and phloem is what ultimately leads to the radial growth.
​
Radial growth brings about large amounts of extremely useful biomass in the form of wood. Wood is the largest terrestrial bio-tissue, an environmental friendly and renewable source of energy for industry and (second to oceans) the largest store of atmospheric CO2. Moreover, the yields of strategic crops for food security depend, directly, on secondary growth. This is the case of cassava and sweet potato.
​
Understanding basic aspects of radial growth
We perform research in Arabidopsis thaliana to understand basic questions related to cellular proliferation, cell identity acquirement and coordination of growth programs, using radial growth as a developmental model system. Through a natural variation and GWAS-guided reverse genetic approach we have recently identified SOBIR1, encoding a LRR-RLK, as a new genetic regulator that controls the precocious formation of xylem fibres, a key cell type in wood. Using genetic and molecular strategies, we have shown that SOBIR1 interacts with ERECTA (another LRR-RLK) to exert its action and that SOBIR1 expression is controlled by the transcription factor BP. Our current focus now is on (i) identifying and characterising further genetic regulators of the process, (ii) understanding new aspects of the hormonal control of it and (iii) using natural variation to ask new questions related with the adaptation value of radial growth. Moreover, by incorporating in our research key species in the evolutionary history of plants, we are seeking to understand essential aspects of how secondary growth arose and evolved in plants.
Understanding root development in cassava: a basic question with biotechnological application.
Cassava in context: the plant that feeds sub-Saharan Africa
The root of cassava is the base of the diet for ~ 500 million people in sub-Saharan Africa (www.fao.org). Its ability to accumulate high amounts of starch in its root, grow in many types of soil and resist severe drought and high temperatures conditions converts cassava into the preferred (and in many cases the only) crop for sub-Saharan breeders and self-sustained farmers. The major problems that cassava is currently facing as a crop are:
1. Reduced nutritional value: despite being an outstanding source of carbohydrates, the cassava storage root does not accumulate enough proteins, vitamins or micronutrients to provide a balanced diet.
2. Post-harvest deterioration: roots of cassava tend to degrade severely a few days after collection.
3. Diseases: many of which entail severe symptoms in the root (for example: cassava mosaic virus leads roots to become corky and non-edible).
4. Threatened yield: due to (i) the expected increased demand as a consequence of population growth and (ii) the increasing interest of the bio-ethanol production industry in its high starch content, the availability of cassava biomass for food might be threatened in the mid-term.
Through our work we aim at generating new biotechnology tools that can provide solid answers to multiple problems in a quick manner.
Cassava root development: two root types in one single system
The cassava root system consists mainly on two types of roots that coexist in every individual: the fibrous roots and the storage roots (Fig 2A). Once roots have been determined as storage, they initially develop as woody roots, which main function is transporting water and nutrients. At a certain point of development and under certain -yet to be determined- signals, a fraction of the woody roots can develop the storage organ by massively proliferating

parenchyma xylem cells that start accumulating starch, becoming, thus, the edible part of the plant. Whether a root remains woody or develops the storage organ depends on the type of secondary xylem that it develops: the woody root produces only xylem fibres and xylem vessels (Fig 2B) while the storage root produces almost exclusively xylem parenchyma cells, (which, as mentioned above, are the cells that store the starch; Fig 2C). Roots that transition from woody to storage undergo a switch in the type of xylem cells that they develop: from fibers and vessels to xylem parenchyma.
What are the genetic and molecular mechanisms that control such xylem cell fate switch or that regulate the woody/storage root ratio are questions that still remain unknown. In this conceptual framework, we use cassava as a model system to understand (i) basic and crucial aspects of the genetics and molecular biology of cell fate determination and (ii) how such determination impacts on organ architecture and function. By generating basic knowledge of the cassava root development, we aim at generating new biotechnology tools to generate new cassava varieties with enhanced capacities for storage root formation (and thus, for yield).